Compression Testing: Exploring Methods, Applications, and Benefits
Compression testing is a vital part of the material science and engineering world. In this article, we will explore what compression testing is, its importance, how to calculate it, and its advantages. We’ll delve into its role in different materials like metallic, polymer, ceramic, and composite materials.
We’ll also detail how data is obtained, what equipment is used, and the specimens required for the test. Additionally, we will explore the standards for obtaining compression testing, examples of materials tested for ISO and ASTM, and its main applications. Finally, we’ll provide additional information and answer some frequently asked questions about compression testing.
In the following text, you will find a summary of the topics that will be addressed in this article.
what is a Compression Testing?
Compression testing is a type of mechanical testing that involves applying a compressive force to a material and measuring its response. The compressive force tends to reduce the size of the material, and the test is designed to determine the material’s behavior under this type of load.
During a compression test, a sample or specimen of the material is placed in a testing machine, where it is compressed between two plates. The machine applies a gradually increasing load to the specimen, and the deformation or change in the material’s dimensions is measured at various points.
This testing method is commonly used to assess materials that are likely to undergo compressive forces in their end-use applications. It allows engineers and scientists to understand the material’s compressive strength (the maximum compressive stress it can withstand), its yield strength (the stress at which it begins to deform permanently), and other properties.
Moreover, compression testing can reveal the material’s behavior under compressive stress, including whether it fails by cracking (brittle failure), deforming plastically (ductile failure), or some combination of the two.
One of the significant benefits of compression testing is that it provides a quantifiable way of assessing a material’s performance under compressive loads. This information can be used in material selection, quality control, research and development, and failure analysis.
It’s essential to note that the specific setup of a compression test (including the shape and size of the specimen, the rate at which the load is applied, and the environmental conditions) can influence the results. Therefore, standard testing protocols, such as those provided by the International Organization for Standardization (ISO) and the American Society for Testing and Materials (ASTM), are often followed to ensure consistency and reliability.
Video about Compression testing
Representation of a Compression Testing
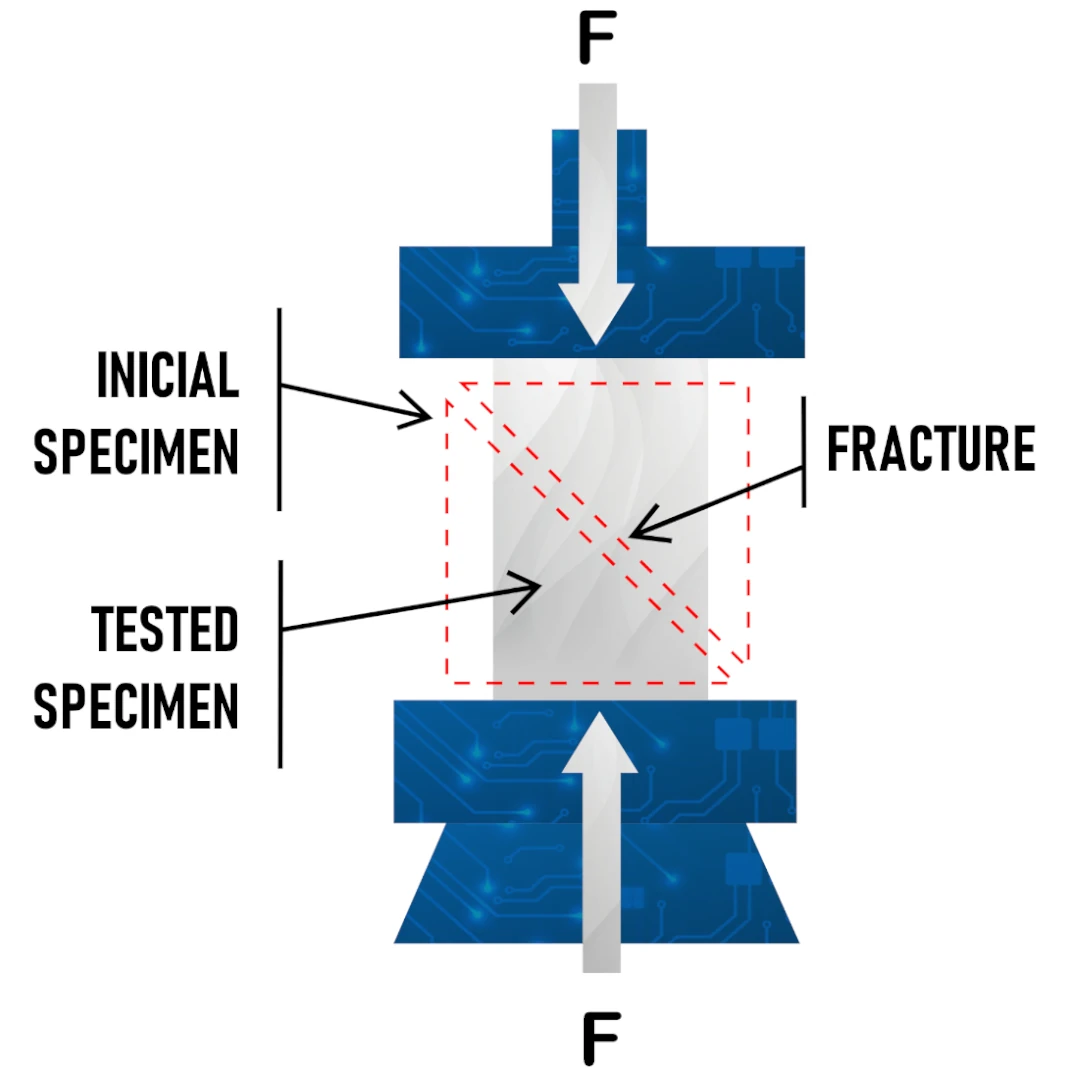
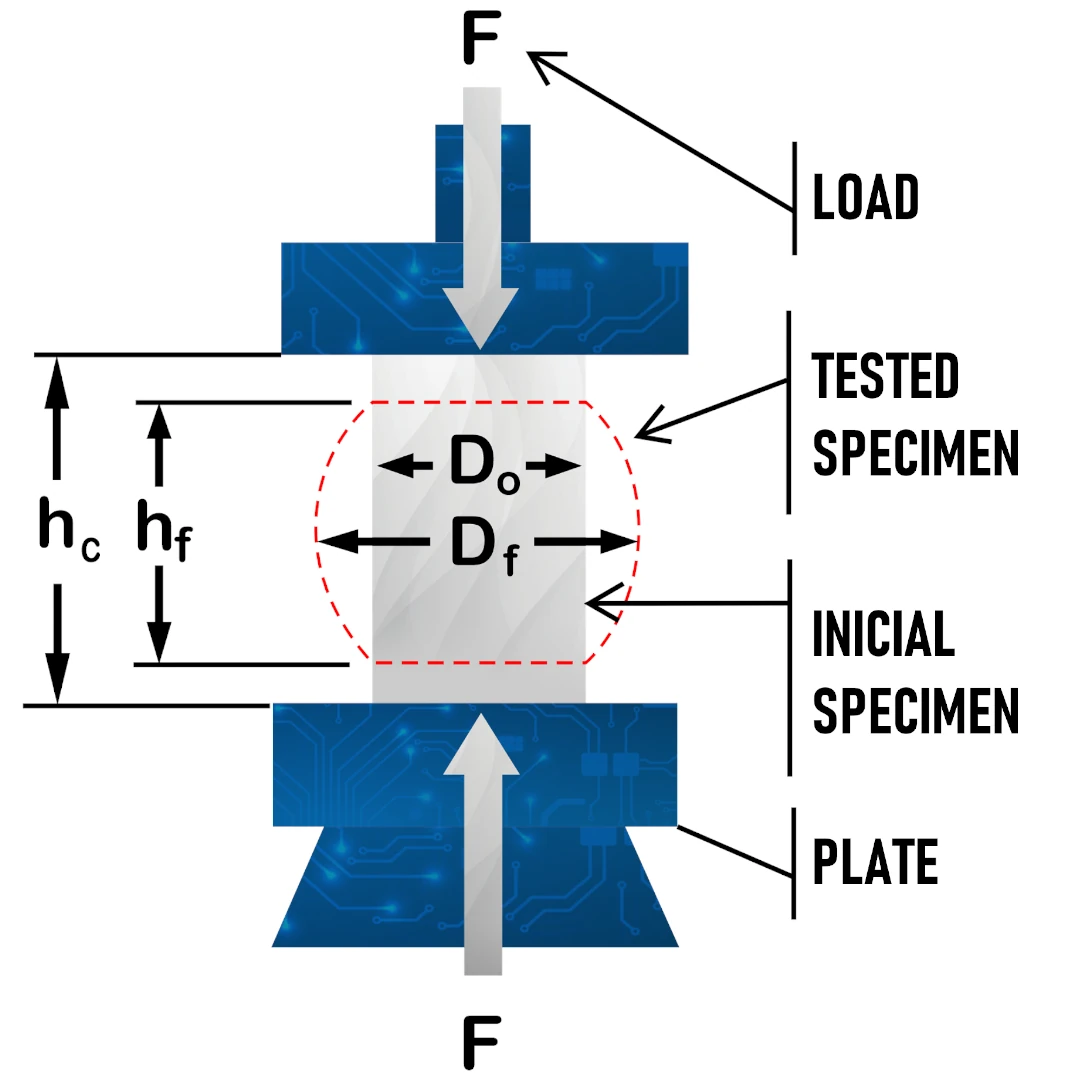
What is the Importance of Compression Testing?
Compression testing plays a crucial role in a wide range of contexts. Here’s why it’s so important:
- Material Selection: Compression testing is essential for selecting materials for specific applications. By understanding how a material behaves under compressive stress, engineers can make informed decisions about whether it’s suitable for a particular use. For instance, a material that exhibits high compressive strength might be chosen for applications that involve heavy loads.
- Quality Control: In manufacturing and production settings, compression testing is a vital quality control tool. It helps ensure that the materials used or produced meet the necessary standards and specifications for their intended use. If a material doesn’t meet the required compressive strength, it could indicate a problem with the production process that needs to be addressed.
- Research and Development: Compression testing is also an important tool in the development of new materials. By testing prototypes under compressive stress, researchers can gain insights into their behavior and make adjustments to improve their performance.
- Failure Analysis: When a product or component fails, compression testing can help determine the cause. By testing a failed part, investigators can determine whether it was unable to withstand the compressive forces it was subjected to in use.
- Safety: Many structures and components must withstand significant compressive forces in their operation, from building materials to car parts. Compression testing ensures these materials can safely bear these loads without failure, protecting people and property.
- Regulatory Compliance: Many industries have regulations that require materials to meet certain compressive strength standards. Compression testing verifies compliance with these regulations.
In conclusion, compression testing is an essential procedure in a wide array of industries, from construction to automotive manufacturing, from aerospace to packaging. By providing a clear measure of a material’s ability to withstand compressive forces, it helps ensure safety, reliability, and effectiveness in numerous applications.
Types of Compression Testing
- Flexure/Bend Testing: Flexure or bend testing involves applying a compressive load to a specimen in a way that induces bending or flexural deformation. This type of testing is commonly used to evaluate the strength and stiffness of materials in bending applications, such as beams or structural components. The specimen is typically supported on two points or across a span, and a compressive load is applied at the center. Flexure testing helps determine properties like flexural strength, modulus of rupture, and elastic modulus.
- Spring Testing: Spring testing focuses on evaluating the compression behavior of helical springs or other types of mechanical springs. The purpose is to measure the spring’s compressive characteristics, such as its load-deflection relationship and spring rate. In this test, the spring is compressed between two platens, and the applied load and corresponding deflection are recorded. Spring testing is crucial for assessing the performance and suitability of springs in various applications, including automotive, aerospace, and industrial fields.
- Top-load/Crush Testing: Top-load or crush testing involves applying a compressive load to a specimen to evaluate its resistance to deformation or failure under a vertical load. This type of testing is commonly used for assessing the compressive strength and stability of materials like packaging containers, bottles, or other products subjected to vertical loads during storage or transportation. The specimen is typically placed under a compression plate or between two parallel plates, and a load is applied until the desired deformation or failure criteria are met.
These different types of compression testing methods are employed based on the specific properties and applications of the materials being tested. By utilizing the appropriate testing technique, engineers and researchers can obtain valuable insights into material behavior, structural integrity, and performance characteristics.
Advantages of Compression Testing
Compression testing offers several significant benefits, making it a valuable tool in material science and engineering:
- Material Characterization: Compression testing provides key data about a material’s mechanical properties, such as compressive strength, yield strength, and modulus of elasticity. This information is crucial for understanding the material’s behavior under compressive loads.
- Quality Control: By determining whether materials meet specified compressive strength requirements, compression testing plays a crucial role in quality control processes. It helps ensure that products are manufactured to the necessary standards, thereby maintaining product integrity and customer satisfaction.
- Material Comparison: Compression testing allows for a direct comparison between different materials. By testing various materials under the same conditions, engineers can determine which material is best suited for a specific application.
- Design Verification: Compression testing can be used to verify that a material or component is suitable for its intended application. For instance, in the design of a new product, compression testing can confirm that the selected material will withstand the expected loads.
- Predicting Material Behavior: The data obtained from compression testing can be used to predict how a material will behave under compressive loads in real-world applications. This predictive capability is essential for ensuring the safety and durability of structures and products.
- Regulatory Compliance: Many industries have regulations that require materials to meet certain compressive strength standards. Compression testing verifies compliance with these regulations, helping companies avoid legal issues and maintain their reputations.
- Cost-Efficient: Compared to some other types of mechanical testing, compression testing can be relatively cost-efficient. The equipment needed is less complex than that required for some other tests, and the test specimens can often be simple shapes like cylinders or cubes.
In conclusion, compression testing is a powerful tool that provides essential insights into a material’s compressive properties, aiding in material selection, design verification, quality control, and more.
Properties Obtained by Compression Testing
Through compression testing, we can gain valuable insights into various mechanical properties of a material. Here are the key properties that can be obtained:
- Compressive Strength: This is the primary result from a compression test. It represents the maximum compressive stress a material can withstand without failure or significant deformation. It’s calculated by dividing the maximum load applied to the specimen by the original cross-sectional area of the specimen.
- Yield Strength: Yield strength is the stress at which a material begins to deform plastically. This means that it won’t return to its original shape once the load is removed. By determining when the material starts to deform during a compression test, we can ascertain its yield strength.
- Modulus of Elasticity (Young’s Modulus): The modulus of elasticity is a measure of a material’s stiffness or its resistance to elastic deformation under load. In a compression test, the modulus of elasticity can be calculated from the initial, linear portion of the stress-strain curve where the material behaves elastically.
- Ductility: Ductility is a measure of a material’s ability to deform without breaking or cracking. The extent of deformation at the point of failure during a compression test can give an indication of the material’s ductility.
- Stress-Strain Curve: The stress-strain curve is a graphical representation of a material’s behavior under compressive stress. By plotting applied stress (load divided by the original area) against strain (change in length divided by the original length), we can observe the material’s response to increasing stress. This curve can provide valuable information about various properties, including the ones mentioned above, as well as others such as the material’s toughness and strain hardening characteristics.
Each of these properties provides a different perspective on how the material behaves under compressive loads, helping engineers and material scientists make informed decisions about the material’s use in various applications.
Calculation Method for Compression Testing
Compression testing involves applying a known load to a material and measuring how it deforms in response. The primary result of a compression test, the compressive strength, is calculated using the following formula:
Stress Calculation:
The stress (σ) is calculated as the applied force (F) divided by the cross-sectional area (A) of the specimen. This gives a measure of the internal forces in the specimen due to the applied load, normalized by the specimen’s size.
Cross-Sectional Area Calculation:
For a cylindrical specimen, the cross-sectional area (A) is given by:
where:
is the diameter of the cylinder.For a cubical or square specimen, the cross-sectional area (A) is given by:
where:
α is the side length of the cube or square.
This cross-sectional area is used to normalize the applied load and calculate the stress on the specimen.
Strain Calculation:
The strain ε is calculated as the change in height Δh divided by the original height ℎ0. This gives a measure of the deformation of the specimen due to the applied load, normalized by the original size of the specimen. In compression testing, the strain is often negative, indicating a reduction in size.
Where:
hf is the final height after the load was applied.
ho is the original height before the load was applied.
Stress-Strain Curve:
The stress and strain calculated at various load levels can be plotted on a graph to create a stress-strain curve. This curve provides a visual representation of the material’s behavior under compression, and can be used to identify key material properties such as the elastic modulus, yield strength, and ultimate compressive strength.
Each of these calculations plays a crucial role in compression testing and provides valuable information about the mechanical behavior of the material under compressive loads.
Calculate conventional stress and real stress
Firstly, it’s important to know that stress is a measure of the internal forces in a material component. It is defined as the force per unit area, and it’s this internal distribution of forces that is responsible for the deformation of the body. In mechanical testing such as tension or compression, we often refer to two different types of stress: conventional (or engineering) stress and true (or real) stress.
Conventional Stress (Engineering Stress): Conventional stress is defined as the applied load divided by the original cross-sectional area of the specimen. This is the most common form of stress that engineers deal with because it’s easy to calculate and understand. It’s important to note that this form of stress does not account for any changes in the cross-sectional area of the material as it deforms. The formula to calculate conventional stress, often denoted as σ, is:
where:
F is the force applied on the material.
A is the original cross-sectional area before any load is applied.
True Stress (Real Stress): True stress, on the other hand, accounts for the change in the cross-sectional area of the material as it deforms. This is a more accurate measure of the stress inside the material, but it’s also more difficult to calculate in practice. The formula to calculate true stress, often denoted as σt, is:
where:
F is the force applied on the material.
Ao is the actual (instantaneous) cross-sectional area under load.
In most cases, the conventional stress is used for engineering calculations because it’s simpler to calculate and the material properties are usually given in terms of conventional stress. However, for large deformations or for materials that undergo significant changes in their cross-sectional area during deformation (e.g., ductile metals), the true stress may provide a more accurate description of the material behavior.
Calculate conventional strain and real strain
Similar to stress, there are also two types of strain, which is a measure of deformation representing the displacement between particles in a material body.
Conventional Strain (Engineering Strain): This is defined as the change in length divided by the original length. This is the most commonly used measure of strain because it’s straightforward to calculate and understand. However, like conventional stress, it does not take into account any changes in dimensions during the deformation process. The formula to calculate conventional strain, often denoted as ε, is:
ε=ΔL/Lo
where:
ΔL is the change in length (the difference between the final length and the initial length).
Lo is the original length before any load is applied.
True Strain (Real Strain): True strain, on the other hand, takes into account the deformation of the material and the change in length that occurs during that deformation. The true strain is defined as the natural log of the ratio of the final length to the original length. The formula to calculate true strain, often denoted as εt, is:
εt=ln(L/Lo)
where:
L is the actual (instantaneous) length under load.
Lo is the original length before any load is applied.
In most engineering applications, conventional strain is used because it’s easier to work with. However, for large deformations, the true strain provides a more accurate measure of the deformation.
Calculate the Compressive Yield Limit
The Compressive Yield Limit (also known as the Yield Strength) is a material property that is defined as the stress at which a material begins to deform plastically. Before the yield point, the material will deform elastically and will return to its original shape when the applied stress is removed. Once the yield point is passed, some fraction of the deformation will be permanent and non-reversible.
In a compression test, the compressive yield limit is determined by steadily increasing the compressive load until the material begins to deform plastically. The yield limit is typically determined using one of the following methods:
- Offset Method: This is the most common method for determining the yield point. A line is drawn parallel to the initial linear portion of the stress-strain curve but offset by a certain amount of strain (usually 0.2%). The point at which this line intersects the stress-strain curve is defined as the yield point.
- Proportional Limit Method: The proportional limit is the point at which the stress-strain curve first deviates from a straight line. This point is often difficult to determine accurately, so it’s less commonly used.
Once the yield point is determined, the compressive yield limit can be calculated as the stress at the yield point. This is typically done by dividing the load at the yield point by the original cross-sectional area of the specimen. The formula is:
σy is the compressive yield limit.
Fy is the force at the yield point.
Ao is the original cross-sectional area of the specimen.
Please note that this calculation assumes that the yield point occurs before any significant change in the cross-sectional area of the specimen. If the cross-sectional area changes significantly before yielding, the true stress at yield might need to be used instead.
Calculate the Compressive Strength Limit
Compressive Strength Limit, also known as Ultimate Compressive Strength, is a key measure of a material’s ability to withstand compressive loads without failure. It is the maximum compressive stress that a material can withstand before it fails or fractures.
In a compression test, the compressive strength is determined by steadily increasing the compressive load until the material fails. The compressive strength limit is then calculated as the maximum stress experienced by the material during the test.
To calculate the compressive strength limit, you use the same formula as for stress, but with the force and area at the point of failure. This can be calculated as follows:
σc is the compressive strength limit.
Fc is the force at the point of failure.
Ao is the original cross-sectional area of the specimen.
The Compressive Strength Limit is calculated based on the original cross-sectional area of the specimen, not the area at the time of failure. This is because it is typically difficult to accurately measure the area at the time of failure, and the original area is generally more relevant for design purposes.
It’s also important to note that while we can calculate the Compressive Strength Limit, the stress at which failure occurs can vary depending on the material and the specific conditions of the test. Some materials, like many metals, have a clear and sudden point of failure, while others, like some polymers or ceramics, may not fail suddenly but gradually deform more and more under increasing stress.
Calculate the transverse dilation
Transverse dilation (or lateral strain) is a measure of the change in dimensions perpendicular to the applied load in a compression test. When a material is compressed, it tends to expand or dilate in the directions perpendicular to the applied load.
The transverse dilation is often calculated using Poisson’s ratio, which is a material property that describes the ratio of the strain in the direction of the applied load (longitudinal or axial strain) to the strain in a direction perpendicular to the load (transverse or lateral strain).
Poisson’s ratio (ν) is defined as the negative ratio of transverse to axial strain. It can be written as:
εtrans is the transverse strain.
εaxial is the axial strain.
If you want to calculate the transverse dilation (or lateral strain), and you know the Poisson’s ratio and the axial strain, you can rearrange the formula to:
εtrans = – ν * εaxial
Remember, the axial strain is calculated as the change in length divided by the original length, similar to the calculation of conventional strain.
Please note that Poisson’s ratio is a material property and is typically determined experimentally. Most materials have a Poisson’s ratio that ranges from 0 to 0.5. For many common engineering materials (like steel), the Poisson’s ratio is about 0.3. Rubber-like materials can have a Poisson’s ratio near 0.5, and some special materials (auxetic materials) can even have a negative Poisson’s ratio.
Also, it’s important to note that Poisson’s ratio assumes that the volume of the material remains constant during deformation, which may not be the case for large deformations or for certain types of materials. In those cases, a more complex analysis might be needed to accurately calculate the transverse dilation.
Similarities and differences between Compression Testing versus Tensile Testing
Both compression testing and tensile testing are fundamental methods of determining the mechanical properties of materials. They provide data that is crucial for design applications, failure analysis, and quality control. While they share some similarities, these two types of tests also have significant differences.
Similarities:
- Purpose: Both tests are performed to understand the mechanical properties of a material, including strength, ductility, elasticity, and yield behavior. These properties are essential for engineers when selecting materials for specific applications.
- Stress-Strain Data: Both tests generate a stress-strain curve, which provides valuable insights into a material’s behavior under different loading conditions. The stress-strain curve includes information about the elastic limit, yield point, and ultimate strength of the material.
- Test Setup: Both tests require the use of a controlled environment, typically provided by a universal testing machine, to apply either a tensile or compressive load to the test specimen. The deformation of the specimen is then measured as the load is gradually increased.
Differences:
- Type of Stress: The most obvious difference is the type of stress applied to the material. In a tensile test, the material is subjected to tension (pulled apart), while in a compression test, the material is subjected to compression (pushed together).
- Deformation Behavior: Materials often behave differently under tensile and compressive stresses. For instance, some materials (like many metals) are more ductile and can undergo more deformation under tensile loads than under compressive loads. Other materials, like ceramics and cast iron, are often more brittle and can withstand higher compressive loads than tensile loads.
- Failure Mode: The mode of failure can also be different in tensile and compression testing. In tensile testing, materials typically fail by fracturing into two separate pieces. In compression testing, failure can occur through a variety of mechanisms, including buckling, cracking, or material crushing.
- Specimen Shape: The shape and size of the specimen can differ between the two tests. Tensile specimens are often thin and long to promote uniform stress along the gauge length, while compression specimens are often cylindrical or cubical to promote uniform stress distribution.
- Strain Measurement: In tensile testing, the elongation of the specimen is measured directly, while in compression testing, the reduction in the height of the specimen is measured. Also, due to the nature of the tests, strain gauges are often used in tensile tests, while this might not be possible in compression tests due to the specimen’s deformation or crushing.
- Poisson’s Ratio: The Poisson’s ratio, which relates the strain in the direction of the applied load to the strain in a direction perpendicular to the load, often has a different meaning in tensile and compression tests. In tensile testing, a positive Poisson’s ratio means that when a material is stretched, it gets thinner. In compression testing, a positive Poisson’s ratio means that when a material is compressed, it gets wider.
In summary, while tensile and compression tests share the same goal of characterizing the mechanical properties of a material, they apply different types of stress, can result in different deformation behaviors and failure modes, and require different specimen shapes and sizes. Therefore, the results of these tests cannot be directly compared and should be used as complementary information when studying a material’s mechanical properties.
Influences of Strain Rate and Temperature on Compression Testing
Strain rate and temperature are key factors that can significantly influence the results of a compression test. They affect both the elastic and plastic behavior of the material being tested, and can dramatically change the material’s response to the applied load. Here’s a closer look at how these factors impact compression testing:
Strain Rate: Strain rate refers to how quickly the deformation or strain of a material occurs. In a compression test, this would be related to how quickly the compressive load is applied or increased.
- High strain rates can increase the apparent strength of many materials. This is because the internal structures of the material (like dislocations in metals) do not have enough time to rearrange themselves and accommodate the deformation. This phenomenon is particularly noticeable in materials that exhibit time-dependent deformation, like many polymers and metals at high temperatures.
- Conversely, at very low strain rates, the material has more time to rearrange its internal structures to accommodate the strain, which can lead to a lower apparent strength.
- Strain rate can also influence the ductility of the material. In some materials, high strain rates can reduce ductility and lead to brittle behavior, while low strain rates can increase ductility.
Temperature: The temperature at which a compression test is conducted can also have a significant impact on the results. This is especially true for materials that undergo phase transformations or have temperature-dependent mechanical properties.
- High temperatures can decrease the strength and stiffness of many materials, as increased thermal motion allows for easier dislocation movement and plastic deformation. This can also increase the ductility of the material and change its failure mode.
- Low temperatures can increase the strength and stiffness of many materials, as decreased thermal motion makes it harder for dislocations to move. However, this can also decrease the material’s ductility and make it more brittle.
- Temperature can also affect the rate of strain hardening and the onset of necking in some materials.
It’s important to note that the effects of strain rate and temperature can vary significantly depending on the specific material being tested. For some materials, these effects may be minor, while for others they can dominate the material’s response. Therefore, it’s crucial to control and record the strain rate and temperature during a compression test, and to consider these factors when interpreting the results.
Compression Testing in Metallic Materials
Metallic materials are often subjected to compression testing to evaluate their behavior under compressive forces. These tests are crucial for applications where the metal will be subjected to significant compressive stresses, such as in structural components of buildings, bridges, and vehicles.
Compression testing in metallic materials involves applying a gradually increasing compressive load to a metal specimen and measuring how much it deforms under this load. The test continues until the metal either fractures or deforms past a specific point.
Here are a few examples of compression testing in metallic materials:
- Steel: Steel is often tested for its compressive strength because of its widespread use in construction and manufacturing. The compressive strength of steel typically ranges from 250-500 MPa, depending on the specific alloy and heat treatment used.
- Aluminum: Aluminum is another metal frequently subjected to compression testing. It’s used in a variety of applications, from aircraft structures to beverage cans, due to its light weight and good strength-to-weight ratio. The compressive strength of aluminum can vary widely but is typically around 95-105 MPa.
- Copper: Copper and its alloys are commonly used in electrical wiring and plumbing due to their excellent electrical conductivity and resistance to corrosion. The compressive strength of copper is typically around 210 MPa.
By understanding the compressive properties of these and other metals, engineers can make informed decisions about their use in various applications.
Compression Testing in Polymer Materials
Polymer materials, such as plastics and rubbers, are frequently subjected to compression testing. These materials are often used in applications where they need to resist compressive forces, such as sealing applications, load-bearing components, and cushioning or impact-absorbing elements.
In compression testing of polymers, a compressive load is applied to a specimen of the polymer material, and its deformation in response to the load is measured. The test continues until the polymer either fails or deforms beyond a certain point.
Here are a few examples of compression testing in polymer materials:
- Polyethylene (PE): This common plastic is used in a variety of applications, including packaging, plastic bags, and pipes. Its compressive strength can vary depending on the specific type of PE, but it generally ranges from 20-30 MPa.
- Polypropylene (PP): PP is widely used in automotive parts, food containers, and carpeting. Its compressive strength is typically around 35-45 MPa.
- Natural Rubber: Natural rubber is often tested for its compressive strength because of its use in seals, gaskets, and shock-absorbing components. Its compressive strength typically falls in the range of 5-15 MPa, depending on its formulation.
By understanding the compressive properties of these and other polymers, engineers can make informed decisions about their use in various applications.
Compression Testing in Ceramic Materials
Ceramic materials are known for their hardness, heat resistance, and electrical insulating properties. However, they are also typically brittle, meaning they can fracture under stress without significant plastic deformation. Compression testing is particularly useful for ceramics because they are often stronger in compression than in tension.
During a compression test on ceramics, a compressive load is applied to a ceramic specimen until it fractures. The maximum load that the ceramic can withstand before fracture gives its compressive strength.
Here are a few examples of compression testing in ceramic materials:
- Alumina (Aluminum Oxide, Al2O3): Alumina ceramics are used in a wide range of applications due to their excellent wear resistance, electrical insulation, and high-temperature stability. The compressive strength of alumina ceramics is typically around 2000-4000 MPa.
- Zirconia (Zirconium Dioxide, ZrO2): Zirconia ceramics, particularly those stabilized with yttria, are known for their high strength and toughness compared to other ceramics. The compressive strength of zirconia ceramics can exceed 2000 MPa.
- Silicon Carbide (SiC): Silicon carbide ceramics are used in high-temperature applications and for their hardness. The compressive strength of silicon carbide ceramics is typically around 2000-3500 MPa.
Understanding the compressive properties of ceramics allows engineers to make informed decisions about where to use these materials and predict their behavior under load.
Compression Testing in Composite Materials
Composite materials, which are made by combining two or more different materials, are known for their high strength-to-weight ratios and versatility. They are used in a wide range of applications, from aircraft and automotive parts to sporting goods and construction materials.
Compression testing in composite materials involves applying a compressive load to a specimen of the composite and measuring its deformation under the load. The test continues until the composite either fails or deforms beyond a certain point.
Here are a few examples of compression testing in composite materials:
- Fiberglass: Fiberglass, which is made by embedding glass fibers in a polymer matrix, is often tested for its compressive strength due to its use in load-bearing structures. Its compressive strength can vary widely but is generally around 100-300 MPa.
- Carbon Fiber Reinforced Polymer (CFRP): CFRP composites, which combine carbon fibers with a polymer matrix, are known for their high strength and light weight. They are widely used in aerospace and automotive applications. The compressive strength of CFRP composites can exceed 1000 MPa, depending on the orientation of the fibers and the specifics of the matrix material.
- Wood-Plastic Composites (WPCs): WPCs, which combine wood fibers or wood flour with a plastic material, are often used in construction applications like decking and fencing. The compressive strength of WPCs can vary widely depending on the specifics of the wood and plastic used, but it generally falls in the range of 20-60 MPa.
By understanding the compressive properties of these and other composites, engineers can make informed decisions about their use in various applications.
Compression Testing in Universal Testing Machine (UTM)
The Universal Testing Machine (UTM), also known as a Universal Tester, Materials Testing Machine, or Tensile Testing Machine, is a versatile piece of equipment that can perform multiple types of mechanical tests, including compression tests. The UTM is designed to test the tensile strength and compressive strength of materials.
The primary components of a UTM include:
- Load Frame: The load frame is the main structure of the UTM. It’s typically a rigid, vertical structure designed to hold the specimen and apply loads. The load frame should be sufficiently stiff and strong to resist bending or twisting during testing.
- Loading Mechanism: This mechanism applies the compressive load to the specimen. This could be a hydraulic actuator, a screw-driven actuator, or an electromechanical actuator, depending on the design of the machine.
- Load Cell: The load cell measures the force applied to the specimen. It typically uses strain gauges that convert the force into an electrical signal, which is then read and recorded by the machine’s control system.
- Extensometer or Deformation Sensor: This device measures the deformation of the specimen under the applied load. There are many types of extensometers, including contact extensometers that physically touch the specimen, and non-contact extensometers that use optical or laser-based systems to measure deformation.
- Test Platens: The platens are the parts of the machine that come into direct contact with the specimen. They apply the compressive load and are designed to be flat and parallel to ensure the load is applied uniformly.
- Control and Data Acquisition System: This system controls the operation of the machine, including the rate of load application, and records the data from the load cell and extensometer. The control system typically includes software that can present the data graphically, calculate material properties, and store the results for further analysis.
In a compression test using a UTM, the specimen is placed between the platens, and the loading mechanism applies a compressive load. The load and deformation are measured and recorded by the control system. The data obtained from the test can then be used to calculate the compressive strength and other properties of the material.
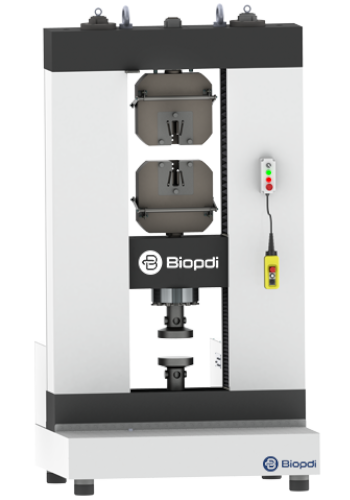
Universal Testing Machine used for compression testing
Obtaining Data from Compression Testing
Compression testing involves applying a compressive load to a test specimen and measuring how it responds. The process of obtaining data from a compression test can be broken down into several steps:
- Specimen Preparation: The first step is to prepare the specimen to be tested. The specimen’s size and shape should be according to the standards for the material being tested. For many materials, a cylindrical or cubical specimen is used. The dimensions of the specimen are measured accurately as they will be used in calculations.
- Test Setup: The specimen is placed between the platens of the compression testing machine. The upper platen is then brought into contact with the specimen.
- Applying Load: The machine applies a compressive load to the specimen. The load is usually applied at a specified rate, which is often set by the testing standard being followed.
- Data Acquisition: As the load is applied, the machine measures the load and the specimen’s deformation. This data is typically recorded at regular intervals throughout the test. In many modern testing machines, the data acquisition is automated, and the machine directly records the load and deformation data.
- Data Analysis: Once the test is complete, the collected data is analyzed to calculate the material’s properties. This can include the compressive strength (maximum load divided by original cross-sectional area), yield strength (load at the onset of plastic deformation), and modulus of elasticity (slope of the initial, linear portion of the stress-strain curve), among others.
The exact process for obtaining data from a compression test can vary depending on the material being tested and the specifics of the test setup. However, the general process involves applying a load, measuring deformation, and using this data to calculate the material’s properties. It’s crucial to conduct the test and analyze the data correctly to ensure accurate and reliable results.
Specimens for Compression Testing
The specimen or test sample is a crucial aspect of compression testing. Its geometry and size can significantly impact the test results, so these are typically standardized for each type of material.
Most compression testing is performed on specimens that are either cylindrical or cubical in shape. The choice of shape can depend on the material being tested and the specific test standard being followed.
- Cylindrical Specimens: These are often used for testing metals and other isotropic materials, which have the same properties in all directions. The diameter and height of the cylinder are measured to calculate the specimen’s cross-sectional area, which is used in calculating compressive strength.
- Cubical or Rectangular Specimens: These are commonly used for testing concrete, ceramics, and some types of polymers. The length, width, and height of the cube or rectangle are measured to calculate the cross-sectional area.
- Other Shapes: For some materials and tests, other shapes might be used. For example, spherical specimens might be used in some types of high-pressure compression tests, and irregularly shaped specimens might be used for testing rocks or other geological materials.
The size of the specimen can also vary depending on the material and the test standard. For example, ASTM C39, a standard for testing the compressive strength of concrete, specifies a cylinder that is 12 inches (305 mm) in height and 6 inches (152 mm) in diameter.
It’s crucial to prepare and measure the specimen accurately to ensure reliable test results. Any flaws in the specimen, such as cracks or surface irregularities, can also significantly affect the results, so the specimen should be inspected and prepared carefully before testing.
In conclusion, the specimen’s geometry, size, and condition are critical aspects of compression testing and can significantly influence the accuracy and reliability of the test results.
Procedure for performing compression testing:
Preparation of Specimen:
- Select an appropriate specimen shape and size according to the testing standard or requirements.
- Ensure the specimen is representative of the material being tested and free from any defects or irregularities.
- Measure and record the dimensions (length, width, height) of the specimen accurately.
Setup the Testing Machine:
- Set up the compression testing machine (such as a Universal Testing Machine) according to the manufacturer’s instructions.
- Ensure the machine is properly calibrated and in good working condition.
- Attach suitable load cell and extensometer to the machine, if necessary.
Platen Preparation:
- Ensure the platens (plates) of the testing machine are clean, flat, and parallel.
- Adjust the distance between the platens based on the specimen’s height and the testing requirements.
Positioning the Specimen:
- Place the specimen carefully between the platens, ensuring it is centered and aligned.
- Apply any necessary alignment aids or fixtures to prevent specimen movement during testing.
Setting Test Parameters:
- Set the desired test parameters, such as the test speed (rate of load application) and the target load or displacement limits.
- Consult the relevant testing standard or specific requirements to determine the appropriate parameters.
Pre-Loading and Pre-Conditioning:
- Apply a pre-load to the specimen to ensure proper contact and alignment between the specimen and platens.
- Perform any necessary pre-conditioning, such as soaking the specimen in a controlled environment or applying pre-stress.
Compression Test Execution:
- Initiate the compression test by starting the testing machine according to the predetermined test parameters.
- Apply a compressive load to the specimen at the specified rate until the desired endpoint is reached (failure, specific deformation, or load).
- Continuously record the load (force) and the corresponding displacement or deformation throughout the test.
Data Collection and Analysis:
- Collect the load and displacement data using the load cell and extensometer or other data acquisition systems.
- Analyze the data to calculate key properties, such as compressive strength, yield strength, modulus of elasticity, and deformation behavior.
Report and Interpretation:
- Compile the test results, including the recorded data, calculations, and any relevant observations or notes.
- Interpret and analyze the results in the context of the specific material, application, and testing requirements.
- Prepare a comprehensive report summarizing the test procedure, results, and any conclusions or recommendations.
It’s important to note that this procedure serves as a general guideline, and specific variations may apply depending on the material, testing standards, and equipment being used. Adhering to recognized standards (such as ASTM or ISO) and following the manufacturer’s instructions for the testing machine is essential for obtaining reliable and accurate results.
Standards for Compression Testing
Let’s explore some of the main ISO and ASTM standards related to compression testing.
ISO Standards
- ISO 604:2002 – Plastics — Determination of compressive properties: This standard specifies a method for determining compressive properties of plastics, including strength, deformation, and strain.
- ISO 844:2014 – Rigid cellular plastics — Determination of compression properties: This standard outlines the method for determining the compressive properties of rigid cellular plastics.
- ISO 1920-10:2009 – Testing of concrete — Part 10: Determination of static modulus of elasticity in compression: This standard provides the procedure for determining the static modulus of elasticity in compression of hardened concrete on test specimens.
- ISO 14126:1999 – Fibre-reinforced plastic composites — Determination of compressive properties in the in-plane direction: This standard specifies the method for determining in-plane compressive properties of fibre-reinforced plastic composites.
- ISO 6784:2009 – Leather — Physical and mechanical tests — Determination of compressive properties: This standard provides the method for determining the compressive properties of leather, including permanent deformation and energy absorbed.
- ISO 9386-2:2000 – Plastics — Determination of compressive yield stress and compressive modulus — Part 2: Compressive tests at high rates of straining: This standard specifies a method for determining compressive yield stress and compressive modulus of plastics at high rates of straining.
ASTM Standards
- ASTM C39/C39M – Standard Test Method for Compressive Strength of Cylindrical Concrete Specimens: This standard outlines the process for determining the compressive strength of cylindrical concrete specimens.
- ASTM D695 – Standard Test Method for Compressive Properties of Rigid Plastics: This standard describes the procedure for determining the compressive properties of rigid plastics, including high modulus composites and electrical insulating materials.
- ASTM E9 – Standard Test Methods of Compression Testing of Metallic Materials at Room Temperature: This standard provides guidelines for compression testing of metallic materials at room temperature.
- ASTM D1621 – Standard Test Method for Compressive Properties of Rigid Cellular Plastics: This standard outlines the test method for determining the compressive properties of rigid cellular materials, particularly expanded plastics.
- ASTM D575 – Standard Test Methods for Rubber Properties in Compression: This standard describes the methods for testing the compressive properties of rubber, both heats and cooled.
- ASTM C773 – Standard Test Method for Compressive (Crushing) Strength of Fired Whiteware Materials: This standard provides a method for measuring the compressive strength of fired whiteware materials.
These standards provide guidelines for conducting compression tests on various materials, ensuring consistency and reliability in the results. It’s essential to follow the appropriate standard for the specific material and application in question.
Materials that are commonly subjected to compression testing
- Concrete: Concrete is a critical material in construction and is often tested for its compressive strength. The compressive strength of standard concrete can range from 20 to 40 MPa (megapascals), but some specialized mixtures can achieve strengths over 100 MPa. In testing, a cylindrical specimen is typically used, and the load is applied until failure occurs, allowing the compressive strength to be calculated.
- Steel: Steel, a commonly used metal in construction and manufacturing, often undergoes compression testing to determine its mechanical properties. The compressive strength of structural steel typically ranges from 250 to 400 MPa. Testing is usually done on a cylindrical specimen, and the load is applied until the specimen deforms plastically, allowing the yield strength and, in some cases, the compressive strength to be determined.
- Polypropylene (PP): Polypropylene is a widely used plastic material known for its versatility. The compressive strength of PP typically falls in the range of 35-45 MPa. In a compression test, a cubical or cylindrical specimen is compressed until it yields or fails, and the yield strength and compressive strength can be calculated.
- Alumina Ceramic (Aluminium Oxide, Al2O3): Alumina ceramics are known for their hardness and are often used in applications that require wear and heat resistance. The compressive strength of alumina ceramics is typically around 2000-4000 MPa. In compression testing, a cylindrical or cubical specimen is loaded until fracture occurs, and the compressive strength is calculated.
These are just a few examples of the many types of materials that can be tested using compression testing. The specific testing procedures and the properties that can be determined can vary depending on the material and the standards being followed.
Applications of Compression Testing
- Quality Control and Assurance: Compression testing is often used in quality control and assurance processes in manufacturing industries. By testing a sample from a batch of products or components, companies can ensure that the batch meets the required specifications for compressive strength, modulus of elasticity, and other properties. Any variations in these properties could indicate issues with the manufacturing process, such as inconsistent mixing or curing.
- Material Research and Development: In the field of material science, compression testing is a critical tool for developing and evaluating new materials. By testing prototypes or samples of new materials, researchers can understand their properties under compressive loads and compare them to existing materials. This can guide the development process and help researchers optimize the material for its intended application.
- Structural Design and Analysis: In structural engineering, understanding the compressive properties of the materials used in a structure is essential for designing the structure to withstand the loads it will face. Compression testing data can inform the selection of materials and the design of structural elements to ensure they won’t fail under compressive loads. For example, in designing a concrete bridge pillar, engineers need to know the concrete’s compressive strength to ensure it can support the weight of the bridge and the vehicles on it.
- Failure Analysis: Compression testing can also be used in failure analysis when a product or component fails under compressive loads. By conducting compression tests on the failed component or similar samples, investigators can identify whether a material’s compressive properties contributed to the failure. This can help identify the cause of the failure and guide efforts to prevent similar failures in the future.
These are just a few of the many applications of compression testing. By providing valuable data on how materials behave under compressive loads, compression testing plays a crucial role in a wide range of fields and industries.
Additional Information about Compression Testing
Compression testing is a versatile and valuable tool in material testing, providing critical data about a material’s behavior under compressive loads. Here are a few additional points to consider about compression testing:
- Stress-Strain Curve: One of the key outputs of a compression test is the stress-strain curve, which shows how the material deforms in response to the applied stress. This curve can provide valuable information about the material’s mechanical properties, including its elastic modulus (the slope of the initial, linear portion of the curve), yield strength (the stress at which the material begins to deform plastically), and compressive strength (the maximum stress the material can withstand).
- Compressive vs. Tensile Properties: While some materials, such as metals, have similar compressive and tensile properties, this is not always the case. For example, ceramics and concrete are much stronger in compression than in tension. Thus, compression testing and tensile testing can provide different, but complementary, information about a material’s properties.
- Effects of Testing Conditions: The results of a compression test can be affected by the conditions under which the test is performed. For example, the rate at which the load is applied can affect the material’s apparent strength and deformation behavior. Additionally, environmental conditions, such as temperature and humidity, can also affect the test results, particularly for materials like polymers that are sensitive to these factors.
- Standards and Precision: To ensure the accuracy and repeatability of compression tests, it’s crucial to conduct the tests according to established standards, such as those published by ASTM and ISO. These standards specify the shape and size of the test specimen, the testing machine’s requirements, the rate of load application, and other key parameters.
- Safety Considerations: Compression testing, particularly when performed on brittle materials that can fracture, can pose safety risks. It’s important to follow safety procedures during testing, including using guards or shields to contain fragments in case of fracture and ensuring that the testing machine is properly maintained and operated.
These are just a few of the many factors and considerations involved in compression testing. By understanding these details, engineers and researchers can make the most of this powerful testing method.
Conclusion
Compression testing is a fundamental method in material science and engineering, used to characterize the behavior of materials under compressive loads. It helps determine critical properties such as compressive strength, yield strength, and the modulus of elasticity, which are essential in various applications from construction to manufacturing and research.
We’ve discussed how compression testing is performed, including the preparation of specimens, the use of a Universal Testing Machine (UTM), and how data is acquired during the test. The importance of adhering to specific standards such as those provided by ISO and ASTM to ensure accuracy and consistency in the testing process has also been emphasized.
Different types of materials, including metals, polymers, ceramics, and composites, were discussed with examples of how compression testing is applied to each. It was shown that the compressive properties obtained can significantly influence the material selection process for specific applications.
The primary applications of compression testing were also covered, highlighting its use in quality control, material research and development, structural design, and failure analysis.
In summary, compression testing is a crucial analytical tool in the field of materials science and engineering, providing essential information that directly impacts the design, application, and performance of materials in real-world applications. It remains an indispensable technique for assessing the quality, safety, and reliability of materials.
FAQ (Frequently Asked Questions)
What is compression testing?
Compression testing is a type of mechanical testing where a material is subjected to a compressive load and its response is measured. This response can be used to calculate properties such as compressive strength, yield strength, and modulus of elasticity.
Why is compression testing important?
Compression testing is important because it provides critical information about a material’s behavior under compressive loads. This information can guide the selection of materials for specific applications, inform the design of structures, and help identify issues in manufacturing processes.
What are some applications of compression testing?
Compression testing is used in a variety of applications, including quality control in manufacturing, material research and development, structural design and analysis, and failure analysis.
What kinds of materials can be tested with compression testing?
A wide range of materials can be tested with compression testing, including metals, polymers, ceramics, composites, and others. The specific procedures and standards used can vary depending on the type of material.
How is a compression test performed?
In a compression test, a specimen of the material is placed in a testing machine, which applies a compressive load to the specimen. The machine measures the load and the specimen’s deformation, and this data is used to calculate the material’s properties.
What is the difference between compressive strength and tensile strength?
Compressive strength is the maximum compressive stress a material can withstand without failure, while tensile strength is the maximum tensile stress it can withstand. Some materials, such as metals, have similar compressive and tensile strengths, but others, like concrete and ceramics, are much stronger in compression than in tension.
What are ASTM and ISO standards for compression testing?
ASTM and ISO are organizations that publish standards for a wide range of tests, including compression testing. These standards provide guidelines for conducting the tests to ensure consistency and accuracy in the results. Examples include ASTM E9 for metals and ISO 604 for plastics.
What is the procedure of compression testing?
The procedure of compression testing involves several steps, including specimen preparation, placement of the specimen in the testing machine, application of compressive load, data acquisition, and data analysis. The specifics of the procedure can vary depending on the material being tested and the testing standard being followed.
See more relevant posts
TENSILE TEST
Tensile testing is a crucial mechanical test used to evaluate the strength and ductility of materials. It involves applying a controlled force. See more
COMPRESSION TEST
Compression testing is a fundamental evaluation method employed to assess a material's ability to withstand compressive forces. See more
SHEAR TEST
Shear testing examines a material's response to forces acting parallel to its surface. By applying a force that causes one part of the material. See more
BEND TEST
Bend testing is a mechanical test that determines a material's flexibility and resistance to fracture under bending forces. See more
FLEXURAL TEST
Flexural testing, also known as the three-point bend test, is employed to measure a material's resistance to bending or flexural stress. See more
FRICTION TEST
Coefficient of friction test measures the amount of resistance between two surfaces in contact when one moves relative to the other. See more
TEAR TEST
Tear test determines a material's resistance to tearing forces, often encountered in applications involving thin films, fabrics. See more
PEEL TEST
Peel testing evaluates the strength of adhesion between two materials, typically an adhesive and a substrate. The test involves separating. See more
BOND TEST
Bond test measures the strength of a bond between two materials. It's used in adhesive and weld test. It helps in determining the reliability of joints and interfaces. See more
INDENTATION TEST
Indentation Force Deflection (IFD) is used to characterize the cushioning or compressive properties of a material, especially foams and soft polymers. See more
FRACTURE TEST
Fracture toughness is a material's ability to resist crack propagation. This property is vital for assessing a material's suitability for applications where structural. See more
PUNCTURE TEST
Puncture test examines a material's ability to withstand penetration by sharp objects or forces. This test to evaluate puncture strength and design materials. See more
CRUSH TEST
Crush resistance test measures a material's ability to resist deformation, particularly in terms of compressive force. See more
TORSION TEST
Torsion testing is a critical method in materials engineering, examining how products and materials behave under twisting forces. See more
AXIAL TORSION TEST
Axial torsion test examines a material's behavior when subjected to simultaneous axial and torsional loads. This test to study shear stress. See more
FATIGUE TEST
Fatigue Testing is the evaluation of a material's endurance and failure under repeated stress and cyclic loading conditions. See more.
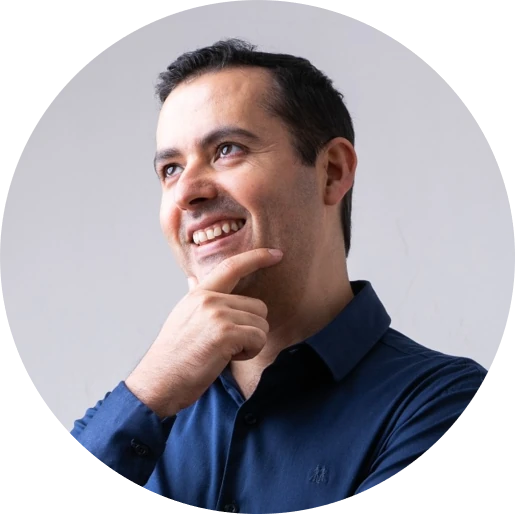
Author: Mardoqueu M. Costa (Entrepreneur, researcher and writer). I have been working for several years in materials science and engineering and biomedical engineering with emphasis on Entrepreneurship. My focus is on the development of equipment, software, mechanical tests, and the evaluation and creation of new businesses. As a researcher and writer, I have contributed to scientific publications and patents, also working as a technical-scientific consultant for several companies. This integrated approach reflects my commitment to innovation and impact in the technology industry.
Contact us
Phones:
+1 (781) 328 - 2010
sales@biopdi.com
Address:
2 Burlington Woods Dr, Burlington, Massachusetts, United States
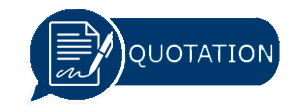
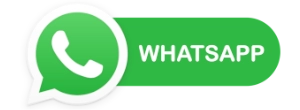